What Is Cellular Manufacturing?
Cellular manufacturing is a production organization method that consists of arranging the shop floor in a way that would accommodate the greatest efficiency. Here are its basic premises, benefits, and disadvantages.
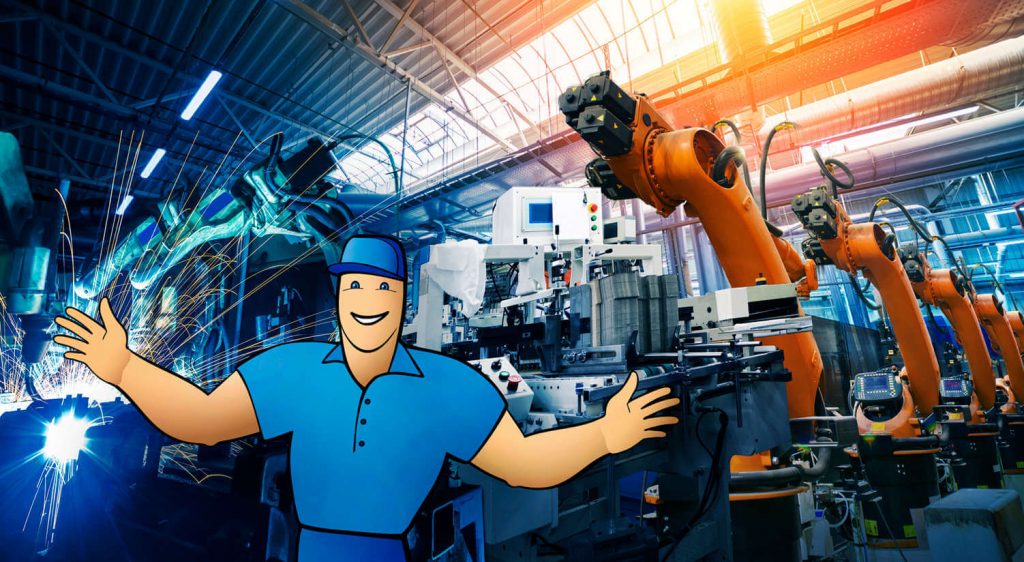
You can also listen to this article:
What is cellular manufacturing?
Cellular manufacturing is a production organization method that arranges workstations and equipment in a sequence that enables products to move through the manufacturing process with maximum velocity while minimizing waste and logistical effort. A subset of just-in-time and lean manufacturing, a cellular manufacturing system aims to organize equipment, machines, parts bins, tools, and workstations in a way that accommodates an optimized flow of continuous production from one cell to the next.
Rather than processing a batch of components in a workstation simultaneously, cells are usually designed to pass parts through one at a time, i.e. in a one-piece flow. This enables the quick production of a wider variety of similar products, i.e. a product family, as individual cells can be adjusted independently without having to rearrange the whole assembly line every time a variation is ordered.
Every single cell is a clearly defined, self-sufficient production unit. Depending on the cell sequence, cells can have specialized workers assigned per cell or be cross-trained to oversee the work of adjacent cells or the whole cell sequence. While a cell may produce finished parts from start to finish, in most cases, cells are arranged in a flow wherein the output of a previous cell is input for the next one.
Most often, cellular production processes are used in job shops and other manufacturing environments that employ a certain degree of customization, including electronics, medical device, aerospace, and automotive manufacturing companies.
History of cellular manufacturing
Cellular manufacturing is not new. Ralph Flanders first envisioned it in 1925. Russian companies took up the concept in the 1930s after Sergei Mitrofanov made others aware of the idea with a book on the subject.
But in the 1970s, cellular manufacturing came into its own as part of a body of initiatives that later became the lean movement. In Japan, cellular manufacturing was instrumental in many lean concepts, including just-in-time (JIT) manufacturing. The idea of an appropriately sized production unit within an optimized ergonomic footprint became critical in reducing waste.
Cellular vs. traditional manufacturing
Cellular manufacturing differs from traditional manufacturing in many key aspects, including:
- Layout. While in traditional high-volume manufacturing environments, production routings are organized in a linear way, most often in long production lines, in cellular manufacturing operations are organized into self-contained and more independent production cells.
- Customization. Traditional production lines are designed to produce standardized products while cellular manufacturing is used to produce products with variations. For example, each work cell could be responsible for a single subassembly used in a product, utilizing families of parts to create variations of the subassemblies.
- Employee involvement. Traditionally, manufacturing employs a hierarchical management structure, with decision-making concentrated at the top. In cellular manufacturing, workers of each production cell are cross-trained and empowered to make decisions that could lead to greater efficiency in their own semi-autonomous work cell.
- Changeover time. In traditional manufacturing, adjusting the production line for different products or product variants is often very time-consuming. In cellular manufacturing, changeover times are significantly reduced as each cell is already specialized to produce variants.
Cellular manufacturing cell designs
Modern-day work cells have become well-engineered and valuable components of manufacturing. Everything from tool selection and its position on the workstation to the number of steps and type of holding bins is carefully considered for inclusion. Many cellular designs are engineered to include a modular approach where workstations or segments can be rolled away and reconfigured for different cells should production needs shift due to seasonality events.
Several considerations go into a sound cell design:
1. Product
The products to be made within the cell must fit a cellular design. Designers need to determine if it is a complete product or a subassembly. If it is the latter, then routings must be included for its upstream and downstream impact.
The production rate for the product should also be determined. If the cell produces all parts within a class of items, it may require an excess capacity to account for spikes in production. On the other hand, if it is one of several cells producing the same parts, then capacity can be tight.
Designers must also take product groupings into account. Often, if the finished goods are completed within the same cell, common parts such as buttons, switches, cords, and housings can be used across several products within the same family. This allows the flexibility of lots as small as one to complete products as a “kit” of similar finished goods.
Also read our Comprehensive Guide to Kitting.
2. Process
It is common for systems engineers to use process mapping to gain a complete understanding of each process step. This helps calculate the number of machines and people and the type of tooling and even parts required for assembly. It also includes optimal lot size and especially the sequence of steps in the process.
3. Logistics
Support structures are also important in cell design. This includes how the cell is scheduled, what containers are used, and how they fit the ergonomics. It also includes how raw materials are brought into the cell and staged for use and how they are removed, and what happens next.
4. Layout
With these considerations in mind, an optimum cellular layout can be determined. The physical structure must integrate with the other cells within the factory and will often be intuitive about what configuration should be used after the above considerations are complete.
Types of cell layouts
There are many types of layouts for configuring cellular manufacturing. These include:
1. Linear
Linear cells (also called “I” cells) are like mini assembly lines. Work proceeds in order, with each machine adding value to the part until it is finished. Linear cells require access to both sides of the layout for optimization. However, it is also possible to configure it with labor on one side and point-of-use raw materials or components on the other.
Example: An example of a linear cell would be rugged metal lunch pails where no steps require repeating, and no equipment is needed to perform more than one task. The material is stamped, bent, buffed, hinged, and painted in order until the unit is complete.
2. Cage
A cage configuration consists of a rough circle or square, usually with one operator inside the “cage.” Products may require multiple passes or repeat passes on each machine several times before completion. In a cage configuration, equipment utilization may be low, and machine use is intermittent based on part design.
Example: An example of a cage would be a CNC machining shop producing sleeved bearing assemblies where the sleeves must be milled or turned to tolerance to fit inside one another, then pressed together, then bored to install grease points, and then pressed again to install the bearing.
3. U-shaped
One of the most common cell designs is the U-shaped cell. Here, all staff and process steps are included within the inside of the “U”. This optimizes cross-training and reduces the time required to produce the part. It also alleviates fatigue and improves communication and collaboration.
Example: An example of a U-shaped cell would be the production of a simple desktop 3D printer. The product would begin with frame riveting and then move to rod and pulley installation. The next step would be the wiring harnesses’ motor installation until the completed unit exits the line. In a U-shaped cell, there may also be other cells of different configurations on the outside of the U. Cage cells could produce the wire harnesses and feed the wire harness assembly section of the U. Another cage may cut and buff rods and install rod bearings to supply that need within the U as well.
4. T-shaped
T-shaped cells are used for production that requires multiple sources of raw materials. Subassemblies are produced on the arm of the “T” and converge into a final leg for final assembly and finish. T-shaped cells work well for semi-finished goods and when different product lines are serviced concurrently.
Example: One example of a T-shaped cell would be custom cosmetics and consumer goods, where finished products may be sold singly or in kits with many iterations. One leg of the T may provide custom initialed soap and the same skin cream, while the other leg produces a unique tray or bag with multiple fill-to-order amenities. The kits then come together in the final perpendicular segment of the T.
5. S-shaped and Z-shaped
S- and Z-shaped cells are often used to work around obstructions. This may be a beam or girder in an older facility with eclectic space to consider. It may also be configured around a heavy machine such as a CNC or metal bending machine where moving it is impractical or impossible. Once in place, S and Z-shaped cells may act like a U or linear cell, depending on the product.
Cell design impacts cycle time, takt time, waste, fatigue, and many other considerations and allows process improvement. Because units may be in quantities as low as one and remain efficient, it reduces work in process and space requirements often overwhelmed with batching.
Implementation process
The work cell implementation process consists of several steps. First, the company’s products must be broken into families. These product groupings may be parts of a similar design that only vary in size, shape, or functionality. Or they may be grouped by the method of manufacturing, such as process step or sequence of tasks.
Second, a production flow analysis (PFA) should occur to group families together. Here, the decision to cluster machines that are complementary to the parts within each family is critical. It helps determine the number of spare parts and raw materials that will be required. Often, the grouping of family parts can reduce SKUs within parts inventory. A single part may be found compatible with another finished product with only a slight modification done by the cell’s equipment.
Finally, optimization of the processes within the cell can occur. This may include prominent elements like step counts between stations within a cell or in the distance from the existing cell to the next cell in the routing.
It may also include planning for material handling, station-to-station product flow, fixed factory costs, and labor costs.
Disadvantages and limitations of cellular manufacturing
Cellular manufacturing is ideal for single-piece flow and small-lot production. But there are limitations and drawbacks:
- Equipment Utilization – In process manufacturing, high-speed automation can outperform tasks that require many precise steps. In cellular manufacturing, equipment within a cell may achieve a low utilization rate. This increases the impact of CapEx ROI on more expensive equipment.
- Maintenance Bottlenecks – In work cells, it is not uncommon for the cell to contain one specific type of machine. If that single piece of equipment breaks, the line is down, and production may bottleneck.
- Administrative Issues – Scheduling and purchasing for a cellular factory is complex compared to process and batch manufacturing. The same is true of MRP systems. Companies may find that the software that works well in a continuous or process production environment lacks the functionality to plan and execute within a cellular environment. Many companies operate hybrid production environments where cells feed traditional production lines. The manufacturing software chosen must be able to accommodate both smoothly.
- Family Group Issues – Just because components may appear similar does not mean they are interchangeable. This may mean that setup times may still create a lag in efficiency even when parts are closely matched.
- Badly Designed Cells – Cells that are poorly designed either in shape or lack of deep understanding of process steps can increase inefficiency and waste rather than eliminate it.
Advantages of cellular manufacturing
Cellular manufacturing also has many advantages. If meticulously designed and optimized for process, and if executed correctly in implementation, a work cell can deliver many benefits. These benefits include:
- Improved communication between workers.
- Reduced changeover times.
- Shortened lead times between order placement and delivery.
- Greatly reduced Work-in-Process inventory.
- Reduced space requirements resulting in less space rented or owned.
- Vastly reduced waste both in production capacity as well as in material resources.
- Quicker identification of defects due to the lack of “buffered” upstream production, leading to greater quality control and higher product quality.
- Easier implementation of zero-defect strategies.
- Continuous improvement is ingrained within cellular manufacturing as each production cell seeks to optimize its processes and reduce waste.
Costs related to cellular manufacturing
Due to the disparity in equipment utilization in cellular manufacturing compared to process, continuous, and batch, the capital investment may be higher. However, it is not often to realize significant cost savings. These savings are cumulative across several contributing factors. Overall, labor costs can be reduced as workers walk less. In-plant packaging may be decreased as transportation requirements and staging is brought in line.
Of course, as an essential component of lean manufacturing, work cells can slash costs lost to waste. As cross-training of staff within the cell increases and improves, this too can impact the cost of quality in a positive light.
Finally, with better quality and lead time, brand reputation is enhanced, leading to higher sales and more business. And cells may even help in the iterative process of designing new products that were not thought of before.
Cellular manufacturing is not for every industry. It must be well designed, fully process-mapped, and constantly improved to produce benefits. However, with the right manufacturing software, it can ramp up its benefits and minimize its disadvantages.
Key takeaways
- In cellular manufacturing environments, cells, equipment, machines, parts bins, tools, and workstations are organized in a way that would accommodate an optimized flow of continuous production.
- Each cell in a manufacturing facility is a clearly defined self-sufficient production unit where workers are cross-trained on all the equipment and tools within the cell.
- When designing a cell, one has to consider the product, the process, the logistics, and the layout.
- There are many different work cell layouts that accommodate different requirements, for example, the linear or I-shape, the cage, the U-shape, the T-shape, and the S- or Z-shape.
- The implementation process consists of several steps of planning, product grouping, and consistent optimization efforts.
- When planned and executed properly, adopting a cellular manufacturing approach can improve communication on the shop floor, shorten lead times, reduce WIP, space requirements, and waste, and improve the quality of your products.
Frequently asked questions
An example of cellular manufacturing is an automotive assembly line where different production cells are responsible for specific tasks such as engine assembly, chassis assembly, and interior installation.
Cellular manufacturing is used across various industries, including automotive, electronics, aerospace, pharmaceuticals, consumer goods, and more, where it can be implemented in both large-scale manufacturing facilities and smaller-scale production environments. It is particularly beneficial in high-volume production scenarios or when there is a need for flexibility to accommodate changing customer demands and product variations.
Cellular production involves organizing manufacturing into self-contained cells for the efficient production of different products, while batch production groups similar products together in larger quantities for more efficient processing.
You may also like: Warehouse Organization – How to Set Up a Smooth-Running Warehouse